A team of engineers and scientists in Berkeley Lab’s Engineering and Physics Divisions have conceived, developed, and built a prototype 3D pixelated detection system for DUNE, the Deep Underground Neutrino Experiment.
DUNE is an international flagship experiment focused on neutrinos, the study of which may help researchers understand the mysterious balance of matter and antimatter in the universe. One of the most abundant and least understood subatomic particles, neutrinos have no electrical charge and very little mass, so they rarely interact with matter and are incredibly difficult to detect. In fact, neutrinos pass through most of the universe as light passes through glass, leaving almost no trace. Researchers face the challenging task of observing these amazingly elusive particles by using state-of-the-art detector systems in underground facilities like DUNE.
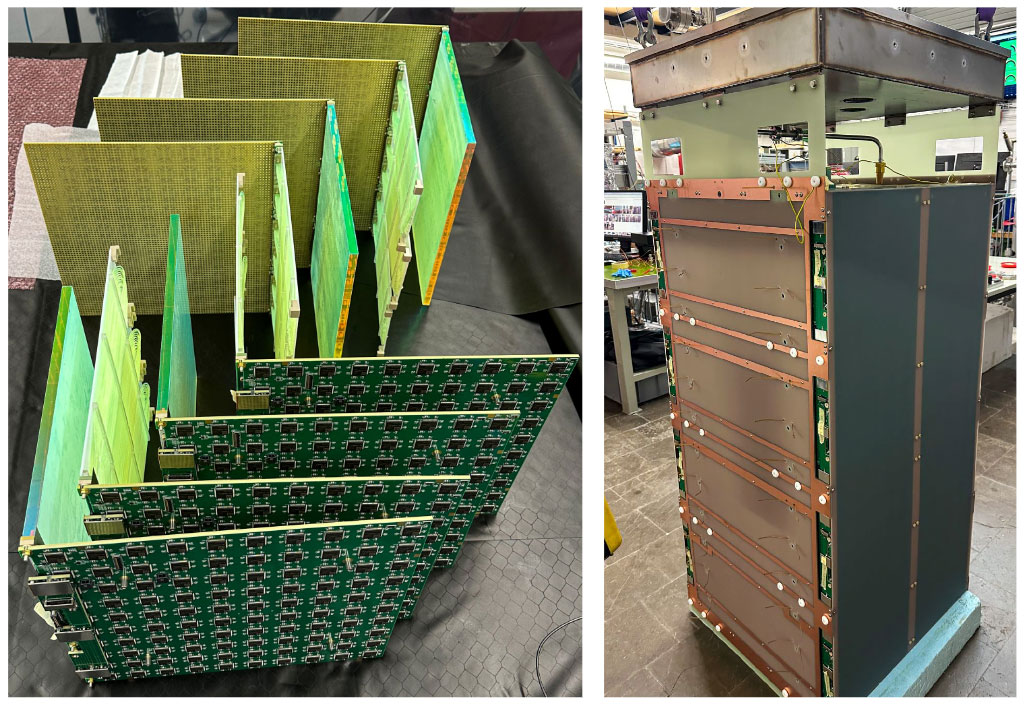
True 3D Readout
New discoveries will be revealed by observing the subtle differences in neutrinos measured by the two sets of detectors that comprise DUNE. One set of detectors will record particle interactions near the neutrino beam’s origin at Fermilab, near Chicago, Illinois. An even larger set of detectors will be built ~1,300 km away – and ~1 km underground – at SURF (the Sanford Underground Research Facility) in Lead, South Dakota.
Berkeley Lab is leading the engineering integration of DUNE’s Near Detector (ND) complex, which will be located in a newly-constructed underground cavern at Fermilab. The key component of the ND is a type of detector known as a liquid-argon time projection chamber (LArTPC) in which the intensity of the neutrino beam produces a large number of simultaneous neutrino-LAr interaction events, causing a “pile-up” of neutrino signals.
To accurately resolve individual neutrino interactions, Berkeley Lab physicists and engineers have invented the LArPix (Liquid Argon Pixel) system, an innovative end-to-end pixelated sensor and electronics system capable of imaging neutrino events in true 3D, a vast improvement upon traditional 2D projective readouts.
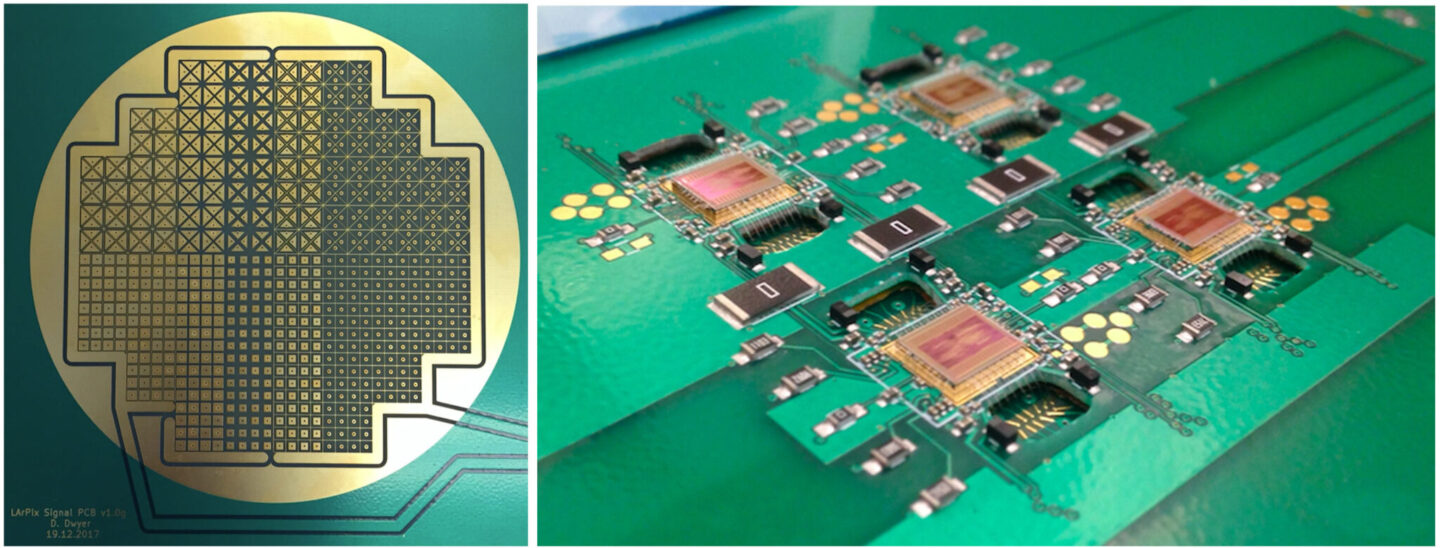
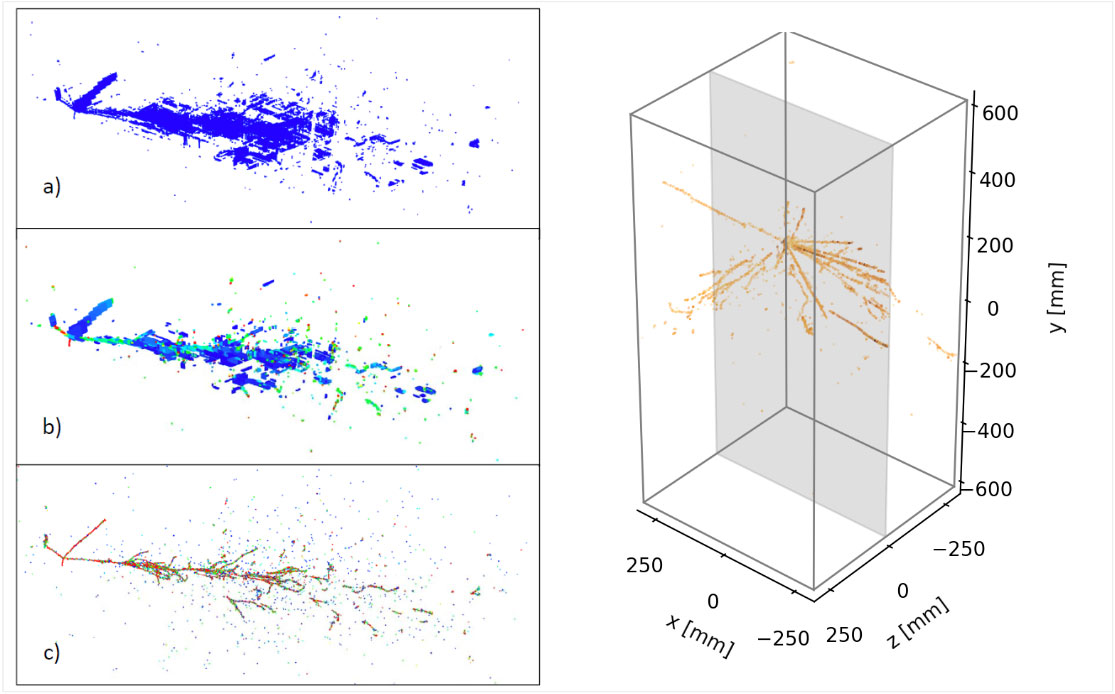
Power efficiency with cryo-compatibility
To handle the large number of neutrino interactions expected in the DUNE Near Detector, Dan Dwyer, the head of the neutrino physics group in Berkeley Lab’s Physics Division and ND_LAr technical lead, conceived of a novel 3D pixelated readout system that could be immersed in a LArTPC. This would provide the ideal medium to measure the neutrino rates as they travel to the far detector site, which employs much larger LArTPCs instrumented with conventional 2D wire plane readout (see figure above).
The team faced several unique engineering challenges on the path to true 3D imaging, which required innovative new sensors that would work effectively and efficiently at the extremely low temperatures required in the detector (~87 K, or -186°C).
The two primary challenges were cryo-compatibility and waste heat. Electronics, particularly integrated circuits, are not typically designed to work at cryogenic temperatures, so custom solutions must be developed and tested to verify that new technologies will tolerate extreme environmental factors while performing accurately.
An enormous number of pixels are required to generate a true 3D readout – approximately 100,000 per square meter. Each pixel in the detector collects the charge generated by neutrino or cosmic ray interactions within the LAr nuclei, and the collected charge is routed to an electronics readout channel, which generates waste heat. Given the very large number of channels, the heat from conventional electronics would boil the argon in the detector, risking high-voltage breakdown and irreversible damage to the detector.
To solve these dual challenges, the Berkeley Lab team developed a novel solution: they designed an early prototype ASIC that implemented cryo-stable transistor behavior and utilized an incredibly power-efficient amplifier that minimized heat while preserving performance (Photo right: Phathakone Sanethavong). The new electronics used less than 100 microwatts per channel, roughly 1,000 times less power than typical detector electronics.
According to Carl Grace, a staff scientist and engineer in the Engineering Division who leads Berkeley Lab’s custom integrated circuit design and development efforts for DUNE, “This project represents Berkeley Lab at its best. Dan Dwyer was facing a difficult problem in Liquid Argon neutrino detectors, namely how to avoid ambiguity in track reconstruction when the event rate was high. Dan had a vision about how to solve it but wasn’t sure it was feasible. He came to the Engineering Division, and after a small pilot study, we jointly determined his idea was highly promising. We then continued this close collaboration throughout the project. The deep application knowledge and vision from the Physics Division, coupled with the creativity, competence, and technical expertise of the Engineering Division, has delivered an innovative solution to a very challenging problem.”
Prototype scalability
With their new sensor prototype in hand – a small version roughly 100 square cm in area and containing ~1,000 pixels – the team then faced the challenge of translating their design into a system that could be implemented at a scale and in a format that could be ‘tiled’ across arbitrarily large detector surfaces.
DUNE’s ND LArTPC is filled with ~280 tons of LAr, requiring ~210 square meters of pixels to cover a surface area corresponding to about 14 million pixels. To mass produce enough pixels on a scale of this magnitude, the team commenced their next stage of research and development with funding from a 2019 DOE Early Career Award.
Instead of relying on manual assembly, the team explored how to cleverly adapt and leverage existing consumer electronics production techniques to produce pixel ‘tiles.’ To isolate the system’s incredibly sensitive pixels (i.e. those able to detect the movement of only a few hundred electrons) from the noisy digital activity across the rest of the tile, the team integrated all of the pixels’ analog and digital transmissions into a single multi-layer printed circuit board. Then, to simplify assembly, all of the active electronics components were merged into the ASIC so that only this element required cryogenic validation.
According to Armin Karcher, the lead engineer for the pixel system, “The key in the design of the pixel tile is the isolation of the top layer, with the digital signals and components from the bottom layer, which acts as a charge-sensitive sensor. Being able to accomplish this isolation, while using only standard printed circuit elements, made it possible to procure the entire core detector as a commodity item. Since industry has spent decades perfecting the scalability of manufacturing electronic assemblies, we are able to move from a small prototype to a large detector relatively easily.”
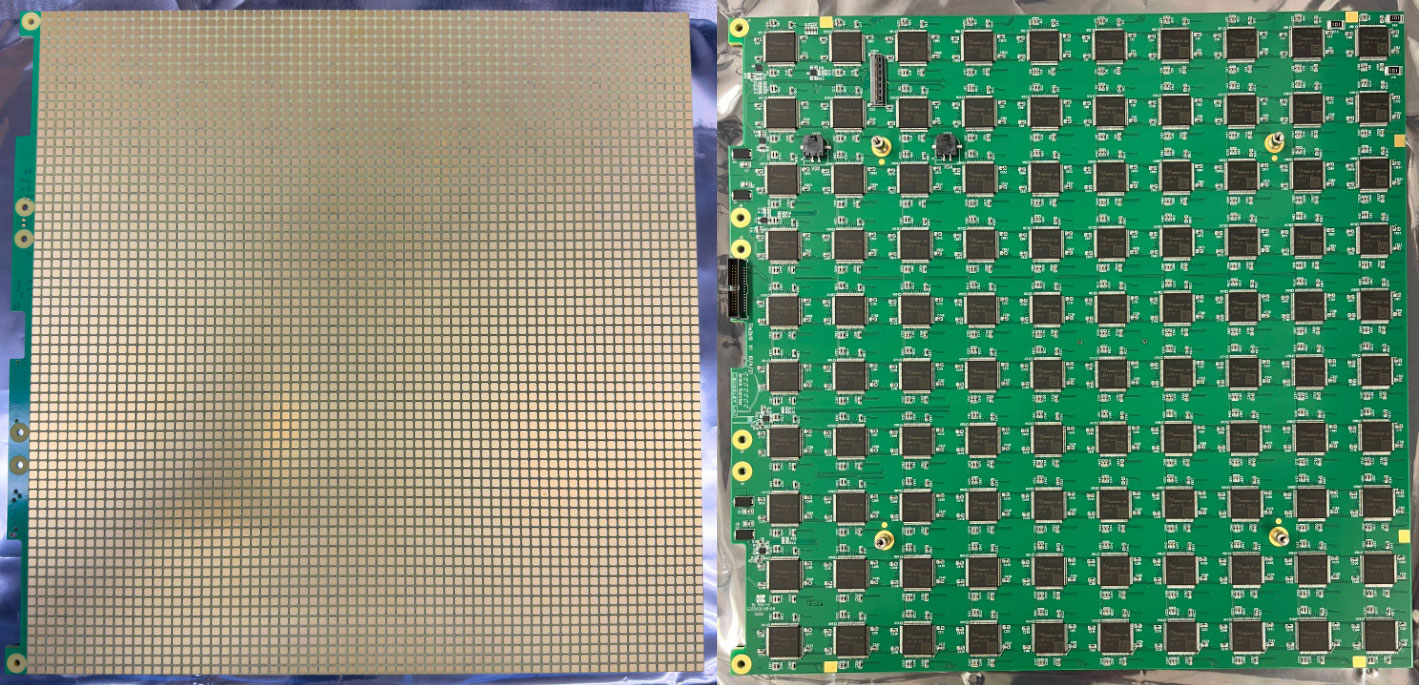
The team also developed Hydra-I/O, a novel adaptable chip-to-chip communication architecture that reduced the risk of single-point failures in the LArPix tile. In Hydra-I/O, the system’s networks can be automatically reconfigured to route around individual failed ASICs without compromising the overall network, which ensures system reliability in extreme environments like the ND. This innovative technology was honored with a 2022 R&D 100 Award.
To probe the efficacy of the new production methodologies, the team fabricated a test LArPix tile with ~5K pixels and tested it in a small-scale LArTPC at Berkeley Lab. During initial testing, the team quickly realized that the tile’s performance exceeded even their best hopes, showing that this was a viable path to build a completely new kind of large-scale particle detector, one with unprecedented performance and providing millimeter-scale 3D granularity at the many-ton scale.
Brooke Russell, an Owen Chamberlain Postdoctoral Fellow in Berkeley Lab’s Physics Division, was one of the first researchers to observe the initial results of the detector: “The raw 3D cosmic ray images that were first detected in the new system were remarkably detailed and high fidelity, and these results immediately demonstrated to us that this was a viable path forward for commercially-produced pixel anodes and control electronics at large scales. LArPix has proven to be a highly performant charge readout technology that produces million-channel systems for multi-ton detectors in a cost-effective way, and this is a game changer for the noble liquid physics detector community.”
From prototype to global research collaboration
Given the promise of these initial results, the team moved quickly to build a large-scale LArTPC as a prototype for the DUNE ND. A team of global collaborators – the ND-LAr Consortium – contributed key elements to the new system’s design and fabrication: several European partners provided light detectors, and the SLAC National Accelerator Laboratory contributed a high-voltage field cage.
Four 1-ton-scale detectors were instrumented with LArPix tiles and assembled at the University of Bern, which hosts the TPC prototyping and co-developed the overall novel LArTPC concept with Berkley Lab. For these four prototype detectors, the Berkeley Lab team produced and tested ~100 LArPix tiles, validating the scalable design and production techniques. Given the affordability provided by industrial production, the team was able to deliver this 0.5-million-pixel system at a small fraction of the typical cost.
The resulting pixel LArTPC prototypes have produced stunning 3D images of cosmic rays with mm-scale granularity (Image right: Dan Dwyer). Combined with the high-performance light detectors, these prototypes represent the most accurate LArTPCs in operation.
Andrew Lambert, the lead engineer for the ND-LAr Consortium, traveled to Switzerland to assist with the assembly and testing of these prototype detectors. “These four detectors represent a significant achievement for the Consortium. These prototypes effectively illustrate the groundbreaking resolution of the LArPix system, and the efforts of the Berkeley Lab engineering and scientific staff were critical in making this endeavor a success.”
Given the impressive performance of these prototypes, the ND-LAr Consortium (which now has 111 members from 39 institutions) is currently assembling all four detectors into a 2×2 array as a multi-ton modular LArTPC, a.k.a. the ArgonCube 2×2 Demonstrator. This will be installed and operated in a high-energy neutrino beam at Fermilab as a demonstration of the ND design, yielding the first neutrino data to be collected by DUNE.
Fabrice Matichard, lead engineer for the DUNE ND, explains, “The DUNE Near Detector is the combination of 3 major sub-detectors: the Liquid Argon Detector, The Muon Spectrometer, and the SAND detector. Each of them has dimensions of approximately 10m x 10m x 10m and weighs over 1,000 tons. In addition, they are equipped with large electric motors so that they can travel across the underground cavern to probe the neutrino beam at different energy levels. This combination of functionality and mobility in these underground detectors requires the DUNE ND engineering team to address unprecedented technical challenges.”
Berkeley Lab leads the engineering team responsible for the design and prototyping of the ND-LAr detector. Berkeley Lab also leads the ND’s cryostat design team, as well as the overall engineering development and installation plan for the DUNE ND Complex to be deployed in 2028-2030.
“Over the past few years we’ve taken 3D LArTPCs from dream to reality, which is extremely fast for particle detector development,” said Dan Dwyer. “We owe this success to the technical strengths and very effective collaboration between Berkeley lab engineers, physicists, and partner institutions. We are very excited about the neutrino data we will collect from the 2×2 Demonstrator over the coming year, and are looking forward to building and operating the DUNE ND-LAr detector later this decade.”
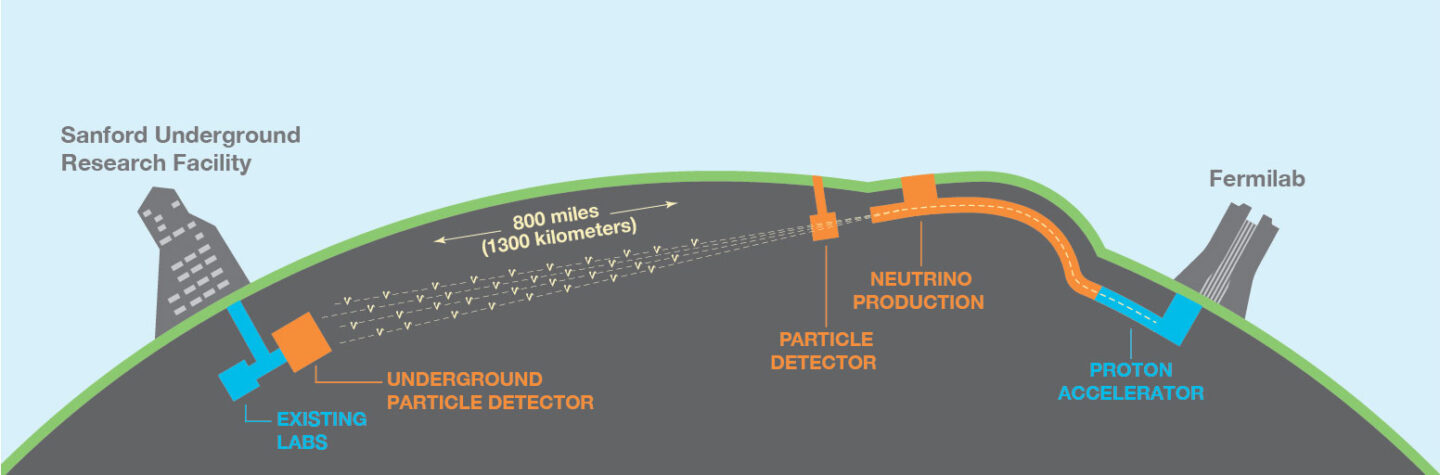
Berkeley Lab is a multiprogram national laboratory managed by the University of California for the U.S. Department of Energy’s Office of Science (DE-AC02-05CH11231). Berkeley Lab Engineering encompasses a broad range of unique expertise areas, collaborating across a global community of multi-disciplinary researchers to develop instrumentation solutions and technical approaches that address critical scientific problems. Current projects include the design and development of innovative semiconductor detectors, custom integrated circuits, mechanical systems, composite technologies, and complex fabrications, among others. For more information and to learn about job opportunities, visit https://engineering.lbl.gov/. Berkeley Lab’s Physics Division conducts a world-leading program that spans a diversity of research areas including cosmology and dark matter, theoretical and particle physics, quantum information science, machine learning, data science and computing, detector R&D, instrumentation, and microelectronics. Berkeley Lab physicists also lead major experiments including the LZ (LUX-ZEPLIN) Dark Matter Detector, DESI (the Dark Energy Spectroscopic Instrument), and CMB-S4, the next-generation cosmic microwave background experiment. For more information, visit https://www.physics.lbl.gov/.