Berkeley Lab researchers have developed new ways to map the distribution patterns of dark matter and intergalactic mass in unprecedented detail, using a combined data analysis of the cosmic microwave background (CMB), and the largest 3-D galaxy map to date released last year by the Dark Energy Spectroscopic Instrument (DESI). Their findings are being announced in a series of new studies that are changing our current understanding of the history of the Universe and the properties of intergalactic matter, as well as how its distribution is affected by the supermassive black holes typically found at the center of galaxies.
“We are combining fantastic new data from DESI, and analyzing it with the latest CMB observations, to get the most out of both,” explains Simone Ferraro, a senior scientist in Berkeley Lab’s Physics Division. “When we integrate the complementary information from both data sets, the results are even more powerful than when analyzed independently, and this opens up a whole new line of research.” Ferraro, who currently leads Berkeley Lab’s research on this topic, initiated early discussions (in 2018) between DESI and the Atacama Cosmology Telescope (ACT), which led to the current research collaboration.
“When we look at galaxies through optical telescopes like DESI, we’re effectively seeing the starlight, which is only a small fraction of the mass of a galaxy,” Ferraro continues. The majority of the galaxy’s mass consists of dark matter, but it also contains a significant amount of gaseous matter, which is considerably less dense and doesn’t emit light, so it’s much more difficult to observe.
Luckily, we have another powerful way to look at the Universe: measurements of the CMB, the leftover heat from the Big Bang that formed very early on in the ancient universe, over 13 billion years ago. “The CMB is usually thought of as the ‘baby picture’ of the universe, showing us what it was like in those very early times,” says Ferraro. “While the CMB itself contains valuable information about the early Universe, here we used it in a whole new way by looking for specific features at the locations of the DESI galaxies. We effectively use the CMB as a ‘backlight’ to look for the ‘shadows’ created by the mass and gas in the DESI galaxies.”
Using a combined data analysis of these two cutting-edge cosmological surveys, the team has developed new and more powerful ways to make detailed measurements that require a unique integration of high-resolution, low-noise observations. Berkeley Lab has a long tradition of leading studies in CMB research, from the pioneering work of George Smoot and his group, who won the Nobel prize for discovering the CMB fluctuations in 2006, to many other subsequent technological and theoretical developments leading into next generation CMB experiments such as the Simons Observatory and CMB-S4.
“These measurements have been very challenging to execute precisely, and our experiments are now advanced enough to detect never-before-seen details about a galaxy’s components and mass.” says Ferraro. This new information is changing the way we look at the history of the Universe and providing researchers with new tools for making these kinds of observations with unprecedented precision.
In two recent papers, a team led by scientists at Berkeley Lab and the University of Pennsylvania (UPenn) used DESI’s 3D galaxy maps with CMB data from ACT to measure the density fluctuations (or “clumpiness”) of dark matter in and around DESI galaxies. Using a technique called gravitational lensing – when galaxies (or other massive objects) bend spacetime and act as a magnifying glass – the team developed new measurements that enable scientists to map all of the different matter components in the Universe, including dark matter. The team’s findings shine new light on the fundamental composition and evolution of our Universe, while supporting the Standard Model of Cosmology, in which the universe is dominated by a mysterious force called dark energy. “We measured how the mass distribution in the Universe has evolved over the past 7 billion years and found excellent agreement with predictions from Einstein’s century-old theory of spacetime,” says Noah Sailer, a graduate student at Berkeley Lab who led the study. This research was conducted by Sailer and Joshua Kim (a graduate student at UPenn), together with Ferraro, Martin White (a senior faculty scientist at Berkeley Lab), and Mathew Madhavacheril (an assistant professor of physics and astronomy at UPenn).
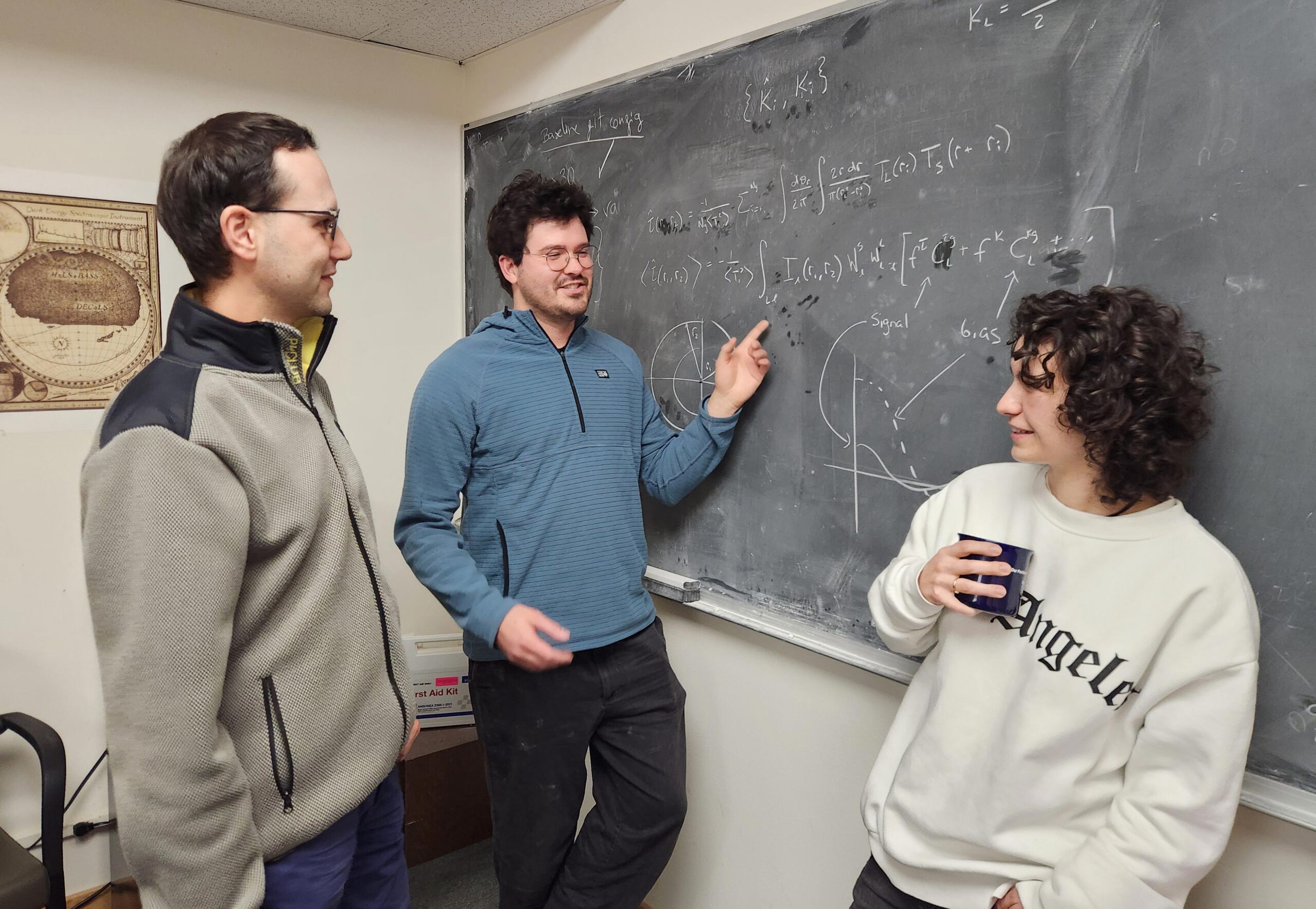
Berkeley Lab researchers (from left) Simone Ferraro, Noah Sailer, and Boryana Hadzhiyska are working on a new analysis technique that combines DESI and CMB data. Photo Credit: Rongpu Zhou, Berkeley Lab
Another study recently looked specifically at the gaseous matter in and around the DESI galaxies. While gases make up about 16% of a galaxy’s mass, and understanding how they are distributed is crucial for making precise cosmological measurements, they are extremely difficult to measure, and most have so far escaped direct observation. In somewhat unexpected results, Berkeley Lab researchers found that galactic gases are distributed far more widely than earlier models had suggested. The results also indicate that the effect of supermassive black holes on intergalactic matter distribution is far more powerful than current theories suggest. These findings will have a profound impact on the interpretation of upcoming cosmological surveys, and in fact, not accounting for these measurements would lead to incorrect results. This research was led by Boryana Hadzhiyska (a joint Miller Postdoctoral Fellow at the University of California, Berkeley, and a Chamberlain Fellow at Berkeley Lab) and Ferraro in collaboration with Bernardita Ried Guachalla and Emmanuel Schaan at the SLAC National Accelerator Laboratory.
In a follow-up study, Hadzhiyska, Ferraro, and Rongpu Zhou (a Berkeley Lab project scientist working on DESI) were able to detect directional variations (anisotropy) in gas density for the first time, showing that the gases are not distributed uniformly in all directions around a specific galaxy, but rather that they are organized in filaments that form a ‘cosmic web’ of matter that permeates the Universe. This new finding represents the next important step towards understanding the connection between the visible and invisible parts of the cosmos.
“We’ve invested several years in developing these new techniques, and what we have published so far is just the tip of the iceberg of what is to come. I’m very excited that this work is finally coming to fruition,” says Ferraro, who is enthusiastic about other new results soon to be reported in other upcoming publications.